par Dr Laurent Schwartz | Mar 20, 2020 | Articles scientifiques, Publications
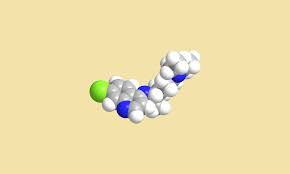
Laurent Schwartz *1,2 , Jean Gabillet1, Ludivine Buhler1,-Marc Steyaert1
1 LIX, Ecole Polytechnique, 93 Route de Saclay, 91128 Palaiseau, France;
2 Assistance Publique des Hôpitaux de Paris (AH-HP), Paris, France
*Correspondence: Laurent Schwartz, École Polytechnique Laboratoire LIX, 91128 Palaiseau, France.
e-mail: laurent.schwartz@polytechnique.edu
Keywords: Hydroxycitrate, alpha lipoic acid, chloroquine, metformin, metabolism, metastatic cancer
Received: 11 October 2014; Revised: 16 October 2014
Accepted: 1 December 2014; electronically published: 3 December 2014
Summary
Background: The combination of hydroxycitrate and lipoic acid has been demonstrated to be effective in reducing murine cancer growth. Early reports suggest a major efficacy in advanced human cancer.
Patients and Methods: Three patients with advanced, chemoresistant cancer were treated by a combination of lipoic acid and hydroxicitrate (MetablocTM) followed by the addition of metformin and chloroquine. Another patient was treated with a combination of Metabloc and cycloserine
Results: Side effects of metabolic treatment are mild. Treatment with Metabloc resulted in a transient drop in tumor marker. The addition of chloroquine and metformin resulted, within one week, in further decrease in tumor marker level. Whether this drop in marker will be sustained is an open question.
These results are in line with published animal data. They also put in evidence the extreme reactivity and variability of cancerous cells. Randomized clinical trials are being defined to confirm the major efficacy of metabolic treatment.
Read more…
par Dr Laurent Schwartz | Sep 19, 2019 | Publications
La médecine déclare de façon récurrente depuis plus d’un siècle que le problème posé par le cancer du sein est en voie d’être réglé.
En 1894, Halsted, un chirurgien nord américain de renom, a prétendu être en mesure de guérir le cancer du sein grâce à une intervention qui porte son nom.
Pour être sûr d’être efficace, il retirait la totalité du sein atteint, les plans musculaires sous jacents et l’ensemble des chaînes ganglionnaires drainant le sein. Selon lui, il fallait anticiper une éventuelle extension de la maladie, laquelle était perçue comme évoluant progressivement, de façon linéaire dans le temps, par étapes successives inéluctables. Elle était d’abord locale puis se généralisait avec des métastases qui conduisaient à la mort. Il fallait interrompre le plus tôt possible ce mécanisme infernal, ce qui justifiait cette véritable mutilation. Son intervention n’était pas nouvelle, l’ablation du sein était pratiquée depuis l’Antiquité et, au XVIIIème siècle, Bernard Peyrilhe préconisait déjà une chirurgie extensive avec ablation du muscle pectoral et des ganglions de l’aisselle. Halsted a amélioré la technique. Avant lui, les patientes mourraient très souvent des suites de l’intervention et l’on ne pouvait pas juger de l’efficacité de celle-ci. Avec Halsted et les progrès de l’asepsie et de l’anesthésie, les patientes survivent plus longtemps, ce qui permet d’apprécier l’effet de la chirurgie sur l’évolution de la maladie.
Ainsi pour Halsted, la taille de la tumeur au moment du diagnostic et l’étendue de l’exérèse conditionnent le pronostic, petit égale précoce et précoce égale curable. On postule l’existence d’une phase suffisamment longue pendant laquelle il serait possible de guérir la maladie.
Tout retard de diagnostic porte préjudice à la patiente.
Le sein est pour ce schéma l’organe idéal, un modèle pur en cancérologie. En effet c’est un organe externe, non vital, susceptible d’une ablation totale, accessible à la vue et au toucher, pour lequel le diagnostic de petites tumeurs est possible. Tous les ingrédients sont apparemment réunis pour que les femmes ne meurent plus prématurément d’un cancer du sein.
Or contrairement aux affirmations d’Halsted son intervention a été un échec.
Elle ne donne pas de meilleurs résultats qu’une chirurgie conservatrice et la mortalité par cancer du sein n’a cessé de croître en France jusqu’aux années 90 malgré les « progrès de la thérapeutique » qu’ont été la radiothérapie, la chimiothérapie et bien que la taille des tumeurs ait diminué régulièrement à partir des années 70.
Au lieu de rechercher les causes de cet échec, de remettre en question cette conception linéaire par étapes de la maladie, nous avons persisté dans l’erreur en adaptant nos pratiques avec une chirurgie plus conservatrice mais en gardant le même schéma théorique de l’histoire naturelle de la maladie :
C’est dans ce contexte qu’intervient la mammographie dans les années 50/60.
Elle apparaît comme l’outil idéal dans cette conception. Elle donne l’espoir d’accéder au diagnostic de tumeurs avant qu’elles n’aient une expression clinique. En l’absence d’une prévention primaire connue et en raison de la grande fréquence de cette maladie, l’idée d’un dépistage de masse organisé fait son chemin.
La maladie cancéreuse est réduite à une image.
Avec la mammographie, le monde médical crie à nouveau victoire. « Cette femme a montré ses seins, elle a sauvé sa vie »
Il n’y a plus qu’à …. Le dépistage de masse organisé devient une réalité.
Les résultats ne se sont pas fait attendre.
Dès 2000, la Cochrane, un organisme indépendant d’expertise médicale, montre que, contre toute attente, le fait de dépister ne permet pas de diminuer sensiblement la mortalité.
A ce constat s’ajoute le fait que les formes évoluées de cancer du sein ne diminuent pas, le nombre des mastectomies totales non plus.
Dans le même temps, on observe également le diagnostic d’une efflorescence de cancers in situ, soi-disant la forme la plus précoce du cancer, sans pour autant que le nombre de cancers invasifs baisse.
L’échec est total, rien ne s’est produit comme prévu. Pire, le dépistage, au lieu de régler le problème, le complique.
Le dépistage s’accompagne d’une augmentation du nombre de cancers diagnostiqués. Dans un premier temps, à sa mise en place, c’est normal car on anticipe ainsi le diagnostic de cancers qui se seraient manifestés ultérieurement. Mais ensuite, logiquement, le nombre de cancers dépistés devrait se stabiliser or il continue d’augmenter. Par ailleurs, après quelques années de dépistage, la comparaison entre population dépistée et non dépistée montre toujours un excès de cancers dans la population dépistée par rapport à la population non dépistée.
Ces cancers en excès sont des cancers qui, s’ils n’avaient pas été diagnostiqués, n’auraient causé aucun inconvénient à la femme. C’est ce qu’on appelle le surdiagnostic.
Le dépistage du cancer du sein génère de la maladie chez des femmes bien-portantes, ce qui est un comble pour une opération de santé publique.
Ce concept est étayé par le résultat d’autopsies systématiques ou d’interventions de réduction mammaire à but esthétique. La proportion de femmes porteuses de cancers asymptomatiques y est beaucoup plus importante que prévu.
Si le dépistage n’a pas fait la preuve de son efficacité, ses effets délétères sont bien là.
Les cancers surdiagnostiqués engendrent un surtraitement intolérable du fait des effets secondaires majeurs qu’ils peuvent occasionner.
Ils augmentent aussi le nombre de femmes considérées comme étant à risque ainsi que leur descendance conduisant à une pléthore de mammographies elles-mêmes susceptibles par leur nombre chez des femmes de plus en plus jeunes d’induire des cancers du sein. Le dépistage s’autoalimente.
Le surdiagnostic est par ailleurs pourvoyeur d’illusions. C’est parce qu’il est plus fréquent parmi les petites tumeurs sans envahissement ganglionnaire qu’il donne l’illusion de l’efficacité d’un diagnostic précoce, du dépistage et des traitements.
Une fois admis, il permet de comprendre pourquoi, alors qu’on diagnostique des lésions cancéreuses de plus en plus petites, la mortalité ne baisse pas sensiblement avec le dépistage. Et lorsqu’on observe une baisse, elle est identique chez les femmes dépistées et non dépistées.
Quand on a pris conscience de l’existence du concept de surdiagnostic, c’est à dire du diagnostic de cancers histologiquement prouvés mais inoffensifs, alors on comprend que la définition purement histologique ne suffit plus pour définir la maladie cancéreuse potentiellement mortelle.
De nouveaux paradigmes sont nécessaires pour construire une nouvelle théorie de l’histoire naturelle de la maladie qui soit plus proche de la réalité.
Au total, il faut mettre en parallèle bénéfices et inconvénients du dépistage.
Dans le meilleur des cas, admettre une réduction de 15 à 20 % du risque relatif de mourir d’un cancer du sein chez les femmes qui se font dépister revient à un gain en risque absolu de 0,05 %. Ce gain minime aura été obtenu au prix d’une augmentation considérable des biopsies négatives, de traitements potentiellement létaux inutiles, d’alertes anxiogènes. Si on regarde maintenant la mortalité globale qui inclut toutes les causes de décès, le gain dû au dépistage est réduit à néant.
Parmi les informations pour un choix éclairé à donner aux femmes, l’information cruciale est qu’on les invite à une opération de santé publique qui n’a pas fait la preuve de son efficacité. Or une opération de santé publique a une obligation de résultats vis à vis de la population ciblée.
On marche sur la tête quand les pouvoirs publics en sont à inviter les femmes à une concertation citoyenne pour savoir s’il faut continuer le dépistage, alors qu’ils ont déjà légiféré et organisé ce dépistage.
Aujourd’hui le problème n’est plus de démontrer l’inefficacité du dépistage.
Il s’agit de comprendre pourquoi cela ne marche pas. Le cancer du sein n’obéit pas aux règles qui lui ont été prescrites par la médecine et depuis plus d’un siècle on se refuse à en tirer les leçons.
Bernard Duperray
À lire Dépistage du cancer du sein – La grande illusion
À regarder Cancer du sein : le dépistage généralisé est-il un échec ?
par Dr Laurent Schwartz | Mai 31, 2019 | Publications
ESSAIS CLINIQUES ET PROSPECTIVE
LEGISLATIVE : VERS UN « DROIT A L’ESSAI »
POUR LES MALADES
Philippe AMIEL
L’expression « droit à l’essai » a été d’abord utilisée pour désigner la revendication de pouvoir expérimenter sur l’être humain en vertu d’un devoir de participer aux essais biomédicaux qui s’imposerait aux malades. Fondée sur la vieille tradition de l’hôpital-hospice où les pauvres étaient soignés gratuitement, mais prêtaient, dans un système de contrepartie silencieuse, leur corps à l’enseignement et, le cas échéant, à l’expérimentation, ce « droit à l’essai » des expérimentateurs a été, en pratique, plus ou moins effectif en France jusqu’à ce que la loi du 20 décembre 1988 assortisse enfin d’un encadrement précis l’autorisation explicite qu’elle accordait de pratiquer les « recherches biomédicales ». Avant la loi, c’est seulement le droit pénal qui, par des décisions qui furent peu nombreuses, bornait les pratiques lorsqu’elles ne pouvaient vraiment plus être assimilées à des actes médicaux plus ou moins curatifs. La « loi Huriet-Sérusclat » fut une avancée en France parce qu’elle permettait le contrôle social de pratiques qui se déroulaient auparavant dans une semi-clandestinité préjudiciable à la protection des sujets. Par l’institution d’une obligation que les protocoles fussent revus par un comité indépendant (et donc préalablement formalisés – et déjà tout simplement écrits), comme le préconisait la déclaration d’Helsinki depuis 1975, elle fit faire de grands progrès à la qualité des essais pratiqués en France. Les grandes révisions de cette loi d’autorisation et de protection, en 2004 puis en 2012, poursuivent la – lire le document (PDF)
par Dr Laurent Schwartz | Mai 21, 2019 | Publications
Jean François Le Bitoux
Une lecture très personnelle du livre de Laurent Schwartz : La Fin des Maladies ? Une Approche Révolutionnaire de la Maladie, 2019, Les Liens Qui Libèrent
Le livre de Laurent Schwartz est un épisode de plus d’une saga contée dans ses ouvrages précédents dans un accompagnement scientifiquement structuré vers une porte de sortie et une grande bouffée d’oxygène.
Le sous-titre proposé, « Sapere aude ! », provient du livre. Page 17, l’introduction s’intitule « Sortir de l’ignorance » et, page 166, le paragraphe §30: « Le courage d’innover ». Leur traduction actualisée ne peut qu’être : Sapere aude ! Osez savoir ! La devise des Lumières !
Je dois afficher que vétérinaire/chercheur des élevages et des milieux aquatiques et ayant la chance de connaître Laurent Schwartz, il m’arrive d’être plus royaliste que le roi. De fait, le vétérinaire de campagne fait de la médecine métabolique à l’insu de son plein gré pour passer d’une espèce à une autre. Quel que soit l’animal concerné, il ausculte les grandes fonctions (digestion, respiration, immunologie, …) le plus souvent avant qu’une « maladie » ne se déclare. Il ne disposait à l’époque, dans les années 1950/70 que d’une vingtaine de molécules à l’arrière de sa 2CV et il prodiguait des conseils zootechniques avant même d’envisager une thérapie médicamenteuse. Par la suite et à la différence d’un chercheur en laboratoire, la pratique naturelle qui s’impose à toute médecine quelle que soit l’espèce, est qu’il faut renforcer les défenses naturelles et s’appuyer sur les énergies disponibles, pour lutter contre le déséquilibre pathologique et les dysfonctionnements observés, dont les mécanismes ne sont jamais totalement connus : il faut quand même soigner ! Reconnaissons que les animaux réagissent souvent mieux et plus vite que l’espèce Homo dite sapiens.
« Il faut contextualiser avant de hiérarchiser » dit Mona Ozouf, historienne des Lumières. En 2019, il y a encore un côté « Guerre des étoiles » dans la guerre contre les cancers, une autre saga des Jedi contre le mal. A quel épisode en sommes-nous ? J’oserai épisode 9 pour ouvrir un œil neuf sur cette série qui n’en finit pas. Ce livre s’inscrit dans une continuité de livres et d’articles, et dans un accompagnement international qu’il ne faut pas ignorer car il renforce chaque situation. Chacun cherche le soleil et l’essentiel à sa porte en fonction de son expérience. Et chaque expérience personnelle de la cancérogenèse est douloureuse.
En oncologie, le Web est un générique qui déroule sans fin des résultats succès et échecs, de manière continue avec plus de 500 publications par jour sous le mot « Cancer » sur le seul moteur de recherche PubMed. Sans même évoquer les cours, les conférences, les entrevues qui sont toujours riches d’informations, de vécus qu’aucune publication scientifique ne peut apporter. Ne pas prendre en compte ces observations partagées d’un discours de prix Nobel à la presse quotidienne est une erreur.
Personne n’aura jamais tout lu mais cette mémoire disponible permet de circuler dans les recoins les plus reculés de la galaxie Oncologie, en tenant compte des dates et des délais. L’impact des radicaux libres en biologie a été découvert au cours des années 1968/75 même si Henri Laborit y faisait allusion bien avant. Le concept d’homéostasie biologie redox d’une cellule vivante ne remonte qu’à l’an 2000. Ce sont ces paramètres et ces domaines qui structurent l’étiologie de la cancérogenèse et l’efficacité de différents traitements métaboliques. Ils disent qu’en pratique « tout se tient » et qu’il existe différentes voies d’accès à la physiologie. Ces paradigmes sont plus complexes que la biologie classique car précisément ils ne sont jamais indépendants les uns des autres. Les publications les plus récentes découvrent de nouveaux liens et des impacts au quotidien : il est normal qu’ils tardent à s’imposer. Mais il faut soigner sans attendre de résultats définitifs !
Il faut ouvrir un œil exercé, expérimenté des domaines scientifiques encore mal connus, pour y trouver de nouveaux repères et progresser. Lire les contributions de cancérologues depuis une cinquantaine d’années, permet de mieux comprendre les hésitations et les bégaiements de l’histoire : trop d’info tue l’info. Il faut effectivement se remettre en question et choisir des voies d’attaque plus directes et il est difficile de faire rentrer d’anciennes connaissances dans de nouveaux domaines. Il faut pourtant faire cet effort.
Et c’est bien de cela qu’il s’agit. Afficher « La fin des maladies ? », N’est-ce pas une provocation ? Même avec un point d’interrogation ! Et sous-titrer « Une approche révolutionnaire de la maladie », alors que le nombre de malades augmente. Chacun se sent légitimement visé. Mais quelle pathologie est plus paradoxale que « le » Cancer ? C’est devenu en quelques décennies une maladie si multiple qu’on en arrive à des traitements personnalisés. Et s’il y a des millions de malades mais pas des millions de maladies ?
Laurent Schwartz s’inscrit dans une double lignée de médecine générale et de réflexion médico-épidémiologique. La médecine de père de famille comporte une bonne dose d’empathie qui n’est plus de mise dans un contexte administratif et économique prégnant. C’était faire de l’humour bon enfant que d’interroger la médecine « Art ou Science ? ». Pour les plus jeunes « Les Arts » renvoient à l’ingénierie et à l’empirisme d’adaptation nécessaire pour être efficace sur le terrain (et les 4 zarts de Brassens !) et « La Science » à l’approche théorique toujours incomplète des phénomènes observés. La réponse était bien entendu : les deux ! Aujourd’hui la réponse à cette interrogation indiscrète est devenue : ni l’un ni l’autre, mais bureaucratie, administration et finance !
Par-delà l’expérience pratique du Dr. Schwartz, cette réflexion médico philosophique s’inscrit aussi dans la réflexion de Mirko Grmek, épidémiologiste et historien dans les pas de Marc Bloch (L’étrange défaite), qui annonçait une « Troisième révolution scientifique ». (cf. G. Lambert, Vérole, cancer & Cie, La société des maladies, 2009)
L’approche du médecin et du vétérinaire est d’abord une volonté de soulager le malade et d’utiliser les énergies disponibles pour combattre des erreurs d’aiguillage métaboliques reconnues ou encore ignorées. En cela les résultats de la recherche ne sont jamais suffisants ! Il faut donc retourner sans cesse au chevet des malades et écouter l’évolution des maux pour renforcer son combat sans prétendre en « contrôler » tous les mécanismes.
La cancérogenèse est une asphyxie cellulaire devenue chronique qui met en route des engrenages pernicieux qui s’installent d’eux-mêmes au cœur de la vie cellulaire en se déconnectant de tissus voisins : une indépendance cellulaire qui s’isole du reste de l’organisme. La médecine métabolique se propose de traiter cet organisme dans son ensemble, de rétablir les liens qui régulent ces fonctions physiologiques énergétiques malades et de soulager ces dysfonctionnements par différentes techniques à l’efficacité reconnue même si les mécanismes intimes ne sont pas complètement éclaircis comme dans le régime cétogène, l’usage d’acide lipoïque, du bleu de méthylène ou de probiotiques. Et la médecine a aussi constaté l’efficacité de ces pratiques dans différentes pathologie lourdes : c’est ce qui permet d’être totalement confiant sur la démarche suivie et d’encourager le Dr. Schwartz.
Pour mémoire, la découverte de Ignace Semmelweis sur l’efficacité d’une solution de chlore pour prévenir la fièvre puerpérale autour de 1845/50 est resté inaudible pendant plus d’un demi-siècle. C’est en 1915 que cette pratique a été redécouverte sous la forme de la liqueur de Dakin qui fut imposée en chirurgie de guerre par Alexis Carel. Cet oubli s’est installé malgré les progrès microbiologiques des années 1890/95, de Pasteur et Koch et les techniques de cultures de M. Pétri. On peut se demander si le dédain de la médecine de l’époque envers les leçons de M. Semmelweis – « Lavez-vous les mains et la médecine sera plus efficace » – ne serait pas dû au fait qu’on a pu croire que la désinfection était un tour de main pour lutter contre la seule « Fièvre puerpérale », sans autre portée « scientifique » plus générale. Ainsi définir une maladie de manière étroite aurait bloqué les progrès de la médecine pendant un demi-siècle ?
Je n’ai donc aucun doute que cette pratique soit le chemin à suivre en ayant conscience, que le contraire des Lumières c’est-à-dire l’obscurité et l’inertie du droit à l’ignorance ne prime au quotidien. Déchiffrer les codes biochimiques de ces thérapeutiques permettra d’appréhender, de guérir et de prévenir d’autres pathologies lourdes, d’éliminer des « maladies » dont l’appellation provenait d’une autre époque, et de changer d’ère thérapeutique.
Par expérience personnelle cette attitude prévaut encore aujourd’hui dans bien d’autres situations pathologiques observables dans d’autres galaxies terrestres. Les cancers touchent aussi les mollusques et je reviendrai sur un autre épisode de cette guerre des cancers et des « maladies » qui n’en sont pas, dans la galaxie Ecologie !